Launch, entry and on-orbit, and environments. It is, therefore, important to under-stand the basic components of mission critical devices and systems that are on the spacecraft. All space craft have a power supply and an electrical distribution system that supplies all systems and instruments of the spacecraft. The details of the power. ICO or intermediate circular orbit is an example of MEO. GPS satellites are not in Geostationary orbits but instead, they orbit twice for every rotation of the earth at a height of 20, 000 km. Handheld terminals have low sending power are hence use LEO for mobile communication. A low earth orbit is an earth-centred orbit that extends to an altitude of between 160 kilometres and 2,000 kilometres from the earth. LEO satellites move quickly – with an orbital period (the time to complete one orbit of the earth) of 128 minutes or less (11.25 periods per day), and an orbital eccentricity (how much the Kepler orbit.
There are benefits to using satellites in LEO or GEO orbits. Ati agp for mac. The key for network operators is to choose the right satellite design, technology, and orbit for the application and market they serve.
The pace of innovation taking place in the satellite industry right now – from ground technology to launch vehicle innovation and satellite design advances – promises to make this decade more productive than the past several decades. Space-based technology innovation includes small satellites in various orbits, as well as higher-throughput satellites operating in the geostationary (GEO) arc.
Continuing advances in technology, combined with ever-growing demand for broadband connectivity in remote locations around the globe, have led to some high-profile projects which intend to leverage the unique capabilities of satellite – whether in GEO or for some constellations in non-GEO orbits, low earth orbit (LEO) in particular.
There are benefits to using satellites in LEO or GEO orbits. The key for network operators is to choose the right satellite design, technology, and orbit for the application and market they serve. Let’s review some of the key elements of each to clarify this emerging discussion (or re-emerging, as this is a subject that has been addressed previously by our industry):
- Latency: An advantage of lower orbits is that they provide lower latency for time-critical services due to the closer proximity to the Earth’s surface. Opinions differ as to the importance of this factor, as the impact of latency is very much application dependent. For voice applications, GEO satellites have been used for decades – for instance as a backhaul to cellular networks – and well-engineered satellite services with the latest in echo cancellation and IP acceleration provide high-quality voice services that are perfectly acceptable for consumers. Latency matters more for specific, highly interactive online applications, such as online gaming or electronic securities trading. However, for most broadband, media and mobility applications, GEO satellite latency has been shown to have a limited impact on the user experience. This is something we continue to watch as future 4G/LTE networks are deployed. Thus far, progress made on ground software to maximize IP and web-searching efficiency has minimized latency as a concern.
- Coverage: GEO satellites appear stationary from the ground, while non-GEO satellites move constantly with the service being handed off by each spacecraft to the next spacecraft in the constellation. Therefore, a succession of LEO satellites is needed to cover any given region. These are smaller satellites, as being closer to the earth allows them to use less power. In the end, it is a trade between a large constellation of smaller satellites in LEO orbit or a few larger satellites in GEO.
- Efficiency: In this trade, the economics are heavily impacted by the question of geographical efficiency. Since small satellites in LEO orbits are moving all the time around the Earth, they spend most of their orbit over oceans and other unpopulated areas. This geographical inefficiency means that much more capacity must be placed into LEO orbit than what is actually needed to provide service. This must be considered when aggregate throughput numbers of non-GEO constellations are communicated. In contrast, a GEO satellite can permanently focus its capacity over the region of demand. The business case for LEO constellations should therefore be stronger when serving customers across the broadest geographical area possible (i.e., global applications), which should lead to a requirement for more capable payloads that can serve a wider range of applications. However, this requirement is likely to add some level of complexity to the satellite payloads and this will go against the general objective to improve the LEO constellation economics by minimizing the size and cost of each of its many satellites.
- Complexity: Low-orbiting constellations are more complex to operate with more costly ground antennas due to the need for tracking and seamless handoff between several satellites. New phased-array antennas that provide, in effect, an electronic steering capability can help reduce some of the cost of this complexity. However, they will need to be used in a wide range of elevations, and this is a challenging requirement for most phased-array designs.
- Cost: Small satellites are much less costly to manufacture because they require less power and accordingly less hardware overall. However, hundreds of small satellites and gateways are required to ensure an operable constellation. This will definitely offset the cost savings derived from lower manufacturing costs. Conversely, GEO satellites are designed to deliver capacity exactly where it is needed, requiring many fewer satellites – and they also typically have a longer life span than smaller satellites in lower orbits. Given the new level of innovation happening in the market alongside the potential of these new constellations, we are hoping this will spur GEO satellite manufacturers to re-engineer their satellite production cycle which should result in a significant cost reduction and time to market. The promise of “software-defined” satellites, fully reconfigurable in orbit, would contribute to eliminating the non-recurring requirements that have traditionally plagued every satellite program and have been a cause for higher costs and minimal production efficiency breakthroughs. This would not only benefit GEO, but make the entire satellite industry more efficient and competitive over the long-term.
Separately, because each spacecraft in a LEO constellation sees a much smaller portion of the earth than satellites in higher orbits, the number of gateways on the ground needed to connect the users of each LEO satellite to the LEO network is equally very high. The direct result is an increase in complexity, more capital expenditures and higher cost of the overall operations of the required terrestrial infrastructure. Not unlike the user terminals, these gateways will have to track and hand-off service between several moving satellites. This is incredibly complex to operate and increases the likelihood of technical issues over time. On the other hand, large-scale production of identical gateways should help gain efficiencies there as well and reduce the cost of the individual gateway. It is also possible to use inter-satellite links. This is another trade-off as such links between satellites can reduce the cost of the ground segment but increase the cost of the space segment.
- Frequency spectrum: In LEO, frequency coordination between systems is of another order of complexity, as constellations always in movement are by nature covering most of the globe, and therefore overlapping geographically with each other everywhere. Frequency coordination between two systems in the same frequency band in non-GEO orbits will be extremely challenging and is likely to require that operators get into the weeds of operational details in order to make their systems co-exist without traffic degradations. For the part of their orbits crossing the equator, LEO constellations also will need to coordinate with each operator of GEO satellites whose frequency rights have priority – introducing yet another level of coordination complexity for these constellations.
- Time to market and adaptability: Finally, as a single GEO satellite is sufficient to cover a particular region, a communications system in GEO can be deployed progressively, with revenues generated immediately with the first spacecraft and then ramping up step by step as new satellites are placed into orbit. By nature of its design, a significant portion of a LEO constellation will need to be built and launched before any regular service can be provided over any region. This will delay the return on the initial investment by several years. Once a LEO constellation is built it is fixed in performance and services delivered, and all satellites must be identical to each other, as they have at different points of their orbit to fulfill the same functions. To upgrade to higher-performance or alternative services, the entire constellation will need to be replaced. This is not the case in GEO where a single satellite can bring new performance and new technology to a region immediately. In a dynamic world in which innovation is happening at an ever faster pace, this lack of adaptability of constellations is an important risk factor to consider in the trade-off.
Whether it is large or small satellites and whatever orbit is used, a key to our collective future success will require manufacturers to break new ground in terms of production, efficiency, cost and time to market. While small platforms may currently have an advantage on this front, software-defined payloads and many other technologies already being used in the industry are propelling Intelsat and other GEO operators down that very exciting path.
The agility, flexibility and ability to progressively innovate within one’s satellite fleet over time will provide the right economics for a fully managed, end-to-end satellite solution.
Ultimately, what really matters is the nature and quality of service delivered to the customers. LEO constellations can be a complementary, rather than competing, offering to GEO satellites. Therefore, operators will continue to compete to fulfill this goal in the best possible conditions and with the right economics. More innovation and more competition around space-based solutions should be in the interest of the end users and will undoubtedly confirm, broaden and strengthen the appeal of satellite communications as the global broadband delivery solution.
–//–
About the Author: Thierry Guillemin is Executive Vice President and Chief Technology Officer of Intelsat.
ORBITAL PARAMETERS
LOW EARTH CIRCULAR ORBITS

Cocktail for mac serial. A circular orbit is mathematically the simplest orbit, although it can be difficult to achieve exactly in practice. Only one parameter is needed to specify the size of the orbit, and that is the radius r, the constant distance from the centre of the Earth to the satellite.
If the radius of the Earth is denoted by Re, then the altitude or height of the orbit (and the satellite) above the Earth's surface is h = r - Re.
Unfortunately for our simplicity, the radius of the Earth is not a constant, varying from 6357 km at the poles to 6378 km at the equator. If our satellite has a low inclination (ie it spends most of its time near the equator), then we might use the equatorial radius. Otherwise we could use the mean radius of 6371 km. Of course, for high accuracy applications we need to consider the varying shape of the Earth at each point beneath the satellite's orbit.
If we know the period of the satellite (the time it takes to make one complete orbit around the Earth) we can compute its height, and vice versa. We can also compute its orbital velocity (v) and a quantity known as its mean motion, the number of orbits it completes in one day.
Isaac Newton provided the formulae we need for these calculations. Starting with the famous second law (N2) of motion F = ma where F is the force on the satellite, m is its mass and a is its acceleration.
Newton's law of gravitation F = G M m / r2 is the required force that the Earth exerts on the satellite.
Microsoft office 2016 for mac активация. A body moving in a circle is actually being accelerated toward the centre of the circle (by the Earth's gravitational force). The acceleration is referred to as the centripetal (directed towared the centre) acceleration, and the force that produces the acceleration is known as the centripetal force.
/cdn.vox-cdn.com/uploads/chorus_image/image/63908857/Screen_Shot_2019_05_28_at_1.54.36_PM.0.png)
The magnitude of the acceleration is given by a = v2 / r .
If we substitute for the force on the left hand side of N2 and the acceleration on the right hand side we end up with:
- G M m / r2 = m v2 / r
where
- G is the Universal Constant of Gravitation, and
M is the mass of the Earth.
- v = √ ( G M / r )
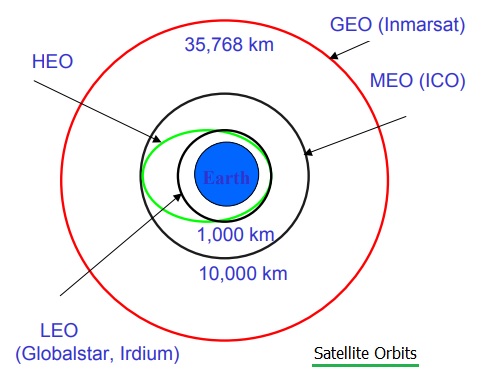
- T = 2 π r / v
- T = 2 π √ ( r3 / (G M ) )
- n = 86400 / T
In this system of units, the values for the gravitational constant and the mass of the Earth is given as:
- G = 6.67259 x 10-11 m3kg-1s-2
M = 5.9736 x 1024 kg
- GM = 3.9860 x 1014 m3s-2
A table of values computed from the above formulae is shown below. Note that the units have been converted into the more typically used quantities before display.
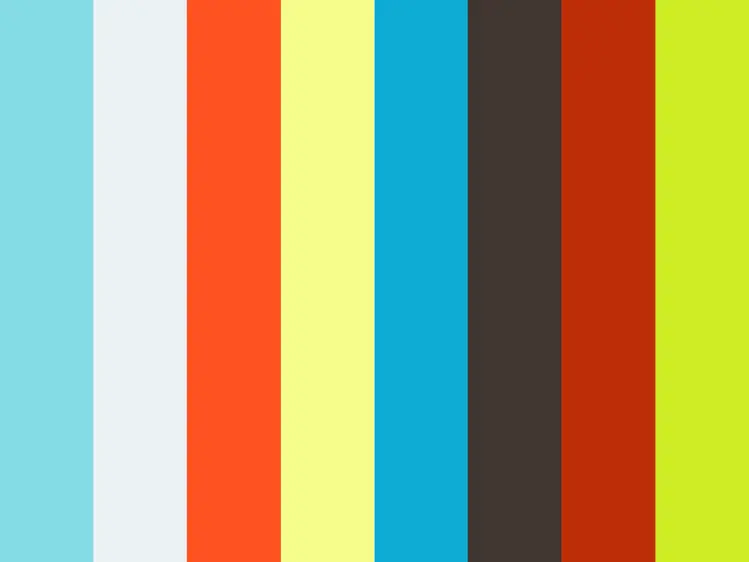
And the figure below displays them in graphical form.
The red curve shows the orbital period in minutes, and its corresponding scale is on the left hand side of the graph. The right hand side scale is used for both the orbital velocity (yellow) which ranges in value from 7 to 8 km/sec, and the mean motion (blue) which varies from 12 to 17 orbits per day.
Leo Orbit
Australian Space Academy
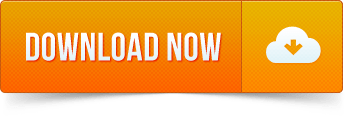
Comments are closed.